|
Fall 2005 - Vol. 6/No. 1
Managing the soil food web in legume-vegetable rotations
by Howard Ferris, Louise E. Jackson, Hideomi Minoshima, Jeffrey P. Mitchell, Sara Sanchez Moreno, Kate M. Scow, Steven R. Temple
These SAFS project researchers study the changes in
soil biology that occur as a result of farming practices. Achieving a functioning soil community
following a history of conventional agricultural practices may require a prolonged transition.
Here researchers describe the importance of soil food webs in alternative farming systems and
explore some approaches to enhancing their activity.
Introduction – Structure, Functions and Importance of Soil Food Webs
The ecological functions of soil food
webs include:
- Decomposition of organic matter
- Cycling of minerals and nutrients
- Reservoirs of minerals and nutrients
- Redistribution of minerals and nutrients
- Sequestration of carbon
- Degradation of pollutants, pesticides
- Modification of soil structure
- Biological regulation of pest species
|
|
The soil food web is that community of organisms that utilize one another,
either by predation or consumption of dead bodies, as sources of carbon and
energy. The activities of soil organisms result in ecological functions
essential to crop production and soil fertility (see box). By consuming,
digesting, assimilating, and metabolizing the bodies of their food sources,
organisms convert complex organic molecules into forms suitable for their
own structural and metabolic needs. Materials indigestible to the consumer
are eliminated in simpler forms that are more accessible to other organisms.
Some of the molecules that are digested may be in excess of the consumer’s
needs and are excreted in mineral forms that are readily available to plants
and to other soil organisms. Molecules taken up by bacteria and passed on
to their consumers are considered to be in the “bacterial decomposition
channel” (Fig. 1). Many of the organisms in this channel are metabolically
very active and molecules pass through the bacterial channel rapidly. Materials
decomposed and digested by fungi are often more complex and their flow through
the “fungal decomposition channel (Fig. 2)” may be slower.
|
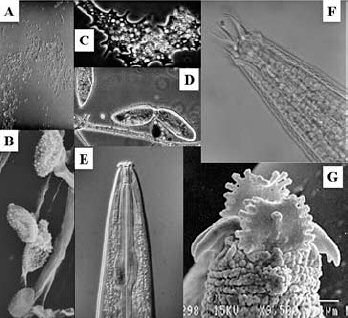
Fig. 1. Organisms of the bacterial decomposition channel. A) Bacteria at the
limit of resolution of a light microscope; B) Bacteria visualized with a scanning
electron microscope; C) and D) Amoeboid and ciliated protozoa; E) Opportunistic
bacterial-feeding nematodes; and F) and G) Bacterial-feeding nematodes with
specialized feeding structures.
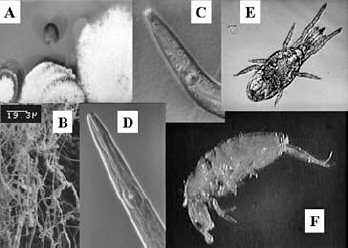
Fig. 2. Organisms of the fungal decomposition channel. A) Fungi under a light
microscope; B) Fungi visualized with a scanning electron microscope; C) and D)
Fungal-feeding nematodes; E) and F) Fungal feeding mite and collembolan.
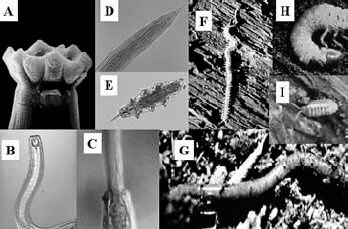
Fig. 3. Organisms sensitive to environmental disturbance and toxic concentrations
of pesticides and fertilizers. Some are predators of organisms at lower trophic
levels, e.g. A, B, C and D. Omnivore and predator nematodes; E) Tardigrades;
others are large bodied and require soil aggregates and channels through the
soil e.g. F, H and I. Larger arthropods and G) Earthworms.
|
Carbon and energy obtained by consumers at the entry level of the food web are
utilized for growth, reproduction and respiration. Carbon dioxide lost from the
soil due to respiration of organisms represents a net loss in resources to the
consumers of those organisms, that is, the next trophic (feeding) level. The loss
of carbon at each trophic level limits the abundance of predators (Fig. 3) that
can be supported by any group of prey. Predators, which may regulate or even
suppress pest species, are usually larger organisms. Their environment is easily
destroyed by physical disturbance of the soil; they are slow to recover from
toxic or environmental perturbations, their life cycles are longer and their
reproductive potential lower than opportunistic organisms at the entry level of
the food web.
Tillage mixes organic matter into the soil so that products of its decomposition
are available to plant roots. However, the disturbance disrupts the higher trophic
levels of the food web. Managing the delivery of resources into the food web
without disturbing its structure in the process, and at the same time optimizing
crop growth, may be the greatest challenge of conservation tillage systems.
A Food Web Management Experiment: Rationale and Approach
We hypothesized that continuous inputs of plant-derived carbon and nitrogen, combined
with conservation tillage (CT), should promote soil communities that decompose
residue and result in a more complex multi-layered food web than in systems with
periodic fallow and standard tillage (ST). The potential for lower yields in crops
grown with CT than ST must be evaluated against the benefits of storing carbon in
the soil and the functions of a complex food web. At the Long-Term Research for
Agricultural Systems (LTRAS) facility at UC Davis, we compared four cropping systems:
- Conservation tillage and continuous crop rotation (CTCC);
- Conservation tillage and fallow rotation (CTF);
- Standard tillage and continuous crop rotation (STCC);
- Standard tillage and fallow rotation (STF).
The continuous crop (CC) rotation had greater plant biomass, more crop cycles, more
continuous plant cover, and more crop diversity than the fallow rotation (F) which
included a fall and summer fallow (Table 1).
Table 1. Continuous crop and fallow rotations under conservation and standard tillage. |
|
Summer 03 |
Fall 03 |
Wint./Spr.03/04 |
Summer 04 |
Continuous Crop |
Tomato |
Sudan/Sorghum |
Garbanzo |
Cowpea |
Fallow Rotation |
Tomato |
Fallow |
Garbanzo |
Fallow |
Although this was a very successful experiment, we experienced some farming problems
with the CTCC systems; the sudan/sorghum cover crop had an inhibitory effect on stand
establishment of the garbanzos where there was a thick residue on the soil. Herbicides
used immediately after planting may have impacted garbanzo growth since a
herbicide-free control plot had higher biomass. Garbanzos in the CT plots were
difficult to harvest because the high density of weeds interfered with cutting the
dried stalks. Cowpeas were originally intended as a cash crop, but since planting was
delayed due to late harvest of garbanzos, the cowpeas became a cover crop. Water
infiltration during the summer irrigation of cowpeas was uneven in the CT treatments
due to accumulation of crop residues.
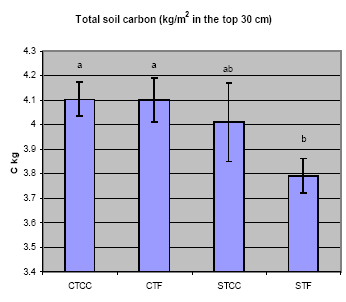
Fig. 4. Total soil C (kg/m2 in the top 30 cm) by treatment. Continuous crop (CC)
and fallow (F) rotations under conservation (CT) and standard (ST) tillage.
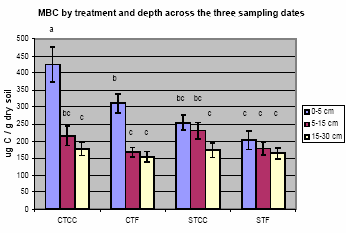
Fig. 5. Total soil microbial biomass C (MBC) by treatment and depth across three
sampling dates (Dec. 03, June 04, Dec. 04). Continuous crop (CC) and fallow (F)
rotations under conservation (CT) and standard (ST) tillage.
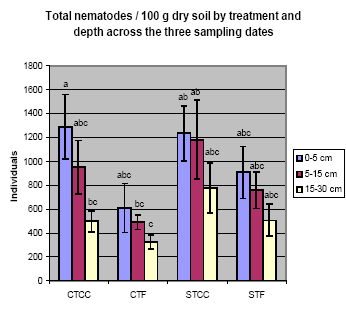
Fig. 6. Total nematodes by treatment and depth across the three sampling dates
(Dec. 03, June 04, Dec. 04). Continuous crop (CC) and fallow (F) rotations under
conservation (CT) and standard (ST) tillage.
|
|
Soil Carbon, Nitrogen, Soil Food Webs: Though total soil C did not increase
after one year of CTCC cropping, increases in total microbial biomass, fungi, and total
nematodes were evident in the surface layer, compared to ST, or CTF. Total soil C
(g/m2 at 0-30 cm) was similar in CTCC, CTF and STCC treatments, and higher than in STF
(Fig. 4).
Nitrate did not differ significantly among treatments but was much higher in June 2004
than on other sampling dates, and higher at 0-5 cm than deeper in the soil, possibly
due to the dry summer conditions that minimized leaching.
The soil microbial biomass (MBC), a good indicator of soil C availability, was
significantly higher at 0-5 cm than below 5 cm. MBC was greatest in the CT plots in
which microbes accumulated in the surface layer presumably due to easy access to
residue on the soil surface (Fig. 5).
Nematodes accumulated in the soil surface layer in all treatments, especially in CT
plots (Fig. 6). The Enrichment Index (EI), which indicates the biomass of opportunistic
fungal- and bacterialfeeding nematodes that respond rapidly to increases in food
resources, was higher in ST plots, especially STCC plots. The Channel Index (CI), an
indicator of the decomposition pathway by bacteria or fungi, was higher in CT than
ST plots, and much lower in STCC than either CT plot, indicating greater activity in
fungal decomposition pathways in CT plots. The EI and CI levels suggest that lack of
disturbance by tillage leads to favorable habitats for fungi and that disturbance by
tillage, with readily available food due to continuous cropping, leads to more
bacteria.
In summary, we explored options for replacing the typical tomato/wheat fallow rotation
of the Sacramento Valley of California with alternative crops, lower inputs of
non-renewable resources, and increased C sequestration. Conservation tillage with
continuous crop rotations of tomato and legumes resulted in lower yields and similar
C storage at 0-30 cm compared to standard tillage with continuous cropping, or
conservation tillage with periodic fallow. Conservation tillage with continuous C
input at the soil surface led to a habitat favorable for microbial biomass, fungi,
and nematodes. However, conservation tillage will require innovative management
solutions to reduce problems such as high weed biomass and uneven water availability
during furrow irrigation.
This research was supported by grants from the Kearney Foundation of Soil Science and CalFed Ecosystem Restoration Program.
• Table of Contents •
|
|